Proteins exhibit astonishing diversity, enabling them to carry out myriad functions within living organisms. Within the human body are tens of thousands of various protein types, each with its unique function. Due to the overall diversity of proteins, scientists initially thought there must be at least 100,000 genes to encode all human proteins. However, researchers broke down our DNA when the Human Genome Project began and found a much humbler number—approximately 20,000 genes. This breakthrough challenged the notion that a higher gene count equals a higher organism complexity, highlighting the enigmatic interplay of genes and the intricate processes that govern protein diversity. But this left researchers with a lingering question: if there are so few genes, how are there many diverse proteins?
The Genetic Script: Transcription and Translation
At the heart of protein diversity is the process of translation, where the information encoded in the DNA is transcribed into messenger RNA (mRNA). This mRNA serves as a template for the synthesis of proteins. The journey begins with mRNA transcription from the DNA template using RNA polymerase. A process called splicing comes into play to generate diverse proteins from the same RNA template. The introns are excised during splicing, and the exons are joined together. This allows for different combinations of exons to be included or excluded, producing distinct mRNA molecules. The resulting mRNA variants, known as isoforms, provide the recipe for synthesizing diverse polypeptides. This mRNA transcript acts as the recipe for protein production in the process known as translation. During translation the mRNA is read by ribosomes and the corresponding amino acids are chained together to form proteins.
Post-Translational Modifications: Glycosylation, Phosphorylation, and Polyprotein Cleavage
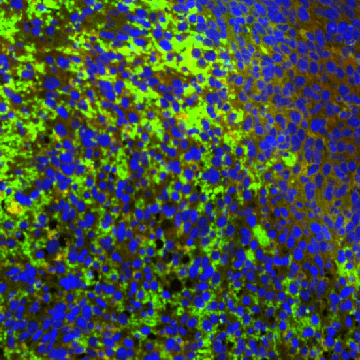
Immunofluorescent Image
The journey from mRNA to functional proteins continues after translation. Post-translational modifications add another layer of complexity, contributing to the incredible diversity of proteins. One common modification is glycosylation, where sugar molecules are added to proteins. A single protein can be glycosylated in many ways; the type of sugar added and where glycosylation occurs can significantly impact a protein’s role and function. This process not only influences the structure and stability of proteins but also plays a crucial role in protein transport, as specific glycosylated tags are needed for proteins to move between the endoplasmic reticulum and the Golgi apparatus of the cell. Another common post-translational modification is phosphorylation, which can activate or deactivate enzymes.
Additionally, some proteins may be derived from larger pro-protein molecules, allowing a variety of small proteins to be made from a much larger precursor molecule. These large molecules, called polyproteins, undergo cleavage to yield smaller, biologically active proteins, including hormones. An example is the prohormone pro-opiomelanocortin, which is cleaved to produce corticotropin and beta-lipotropin, each with its unique function. These post-translational modifications play a critical role in protein structure and function diversity. Proteins can be visualized in cells and tissues using immunohistochemistry (IHC) and immunofluorescence (IF) detection methods.
Harmony of Techniques: Immunohistochemistry (IHC), Immunofluorescence (IF), and RNA-FISH
Due to the diversity of proteins, there are various ways for scientists to visualize and analyze the distribution of proteins within tissues and cells. IHC and IF commonly utilize molecular methods to detect proteins within cells or tissues. The ability to recognize and differentiate between various protein isoforms and modified forms enhances our understanding of cellular processes and aids in identifying disease markers. These techniques allow scientists to visualize what proteins are present in normal tissues to compare protein expression patterns to those of diseased tissues. Adding another note to this symphony is RNA fluorescence in situ hybridization (RNA-FISH). This technique allows researchers to visualize and localize specific RNA molecules within cells, providing a dynamic perspective on gene expression. By combining IHC, IF, and RNA-FISH, scientists can create a comprehensive picture that unveils the intricate relationships between RNA and protein dynamics, enriching our understanding of cellular function and dysfunction.
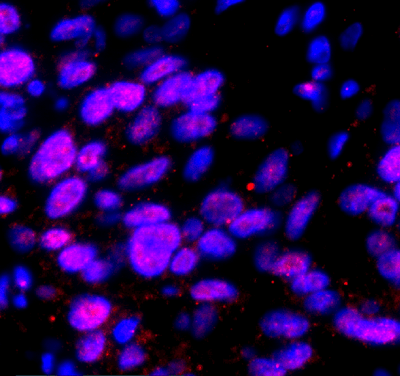
40X imaging of B-actin RNA with HCR RNA-FISH (red) and DAPI (blue)
The Crescendo: Molecular Biology and Medicine Knowledge
The journey from a single RNA template to a vast array of proteins is a molecular ballet involving intricate steps of transcription, splicing, translation, and post-translational modifications. The resulting diversity not only fuels the complexity of life but also forms the basis for advancements in diagnostic and therapeutic applications, particularly in the fields of IHC, IF, and RNA-FISH. This symphony of techniques harmoniously contributes to the crescendo of molecular biology and medicine knowledge.
As a leader in IHC and IF imaging, Visikol provides clients with high-quality data about protein expression within various tissue types. Through a combination of skilled professionals and cutting-edge technologies, Visikol is pushing the boundaries of immunolabeling and imaging, bringing us closer to a deeper understanding of human health and disease. Reach out to a member of our team today to find out more!